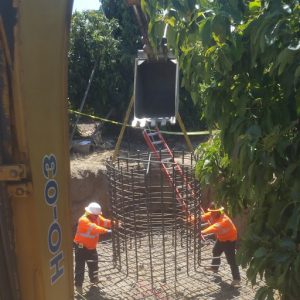
The essential ingredients of concrete batch: By volume, contains roughly 10% cement, 20% water and air, 30% sand, and 40% gravel. The exact proportions vary up or down a little, but 10-20-30-40 is a good rule of thumb. So 70% or more of any concrete structure is nothing but sand and gravel. That’s very cost-effective:Good aggregate is actually stronger than cement, and much cheaper. The main idea when you make concrete is to use as much aggregate as practical, with just enough cement paste to glue the mix together.
To achieve this, you want a “well graded” sand and gravel mix (containing roughly equal proportions of the various aggregate sizes, from large gravel on down to medium or fine sand). That way, the smaller particles fill in the void spaces between the larger chunks, so that there is very little void left to fill with cement paste.
When you pump concrete, the stone shouldn’t be too big – 3/4-inch gravel is a typical maximum for pumped concrete. But if you’re taking your mix straight off the chute, gravel as large as 1-1/2 inches should give you no trouble, and large aggregate is actually a good thing to have. For one thing, it helps reduce shrinkage. A lot of shrinkage happens when water evaporates out of the cement paste – which means the more stone and sand there is, the less overall shrinkage. Also, large chunks of gravel interlock against each other, which helps restrain shrinkage.
There’s another benefit to large aggregate. The larger the particles you use to get well-graded aggregate – the bigger the gravel and the coarser the sand – the less water and cement your mix will require to begin with. This is because it takes less fluid to coat big particles than to coat little particles. A mix that uses less cement is more economical; and other things being equal, lower water demand will mean better concrete.
Types of Cement
We’ll get back to the water, but first let’s consider cement. In the mixing truck, the cement and water form a paste. After you place the mix in the forms, the water and cement react together chemically (hydrate) to form a solid matrix. This hydration reaction (which also generates heat) binds the sand and gravel into a hard mass.
There are five main kinds of cement on the market, known as Type I through Type V. If you include the air-entraining varieties of Types I, II, and III, there are eight types; but air-entraining cements are less common now that there are air-entraining admixtures that we can use instead.
Probably 90% of the cement sold in the U.S. is either Type I or Type II. Standard Type I cement is good for almost every residential purpose, except where soils and ground water contain sulfates that can attack concrete. In sulfate areas (mainly out West), Type II is used because it’s sulfate resistant. Type V, the cement with the highest sulfate resistance, may be necessary where extreme sulfate conditions exist.
Most ready-mix plants don’t stock Type IV cement, and a residential builder would probably never need it. This “low heat of hydration” cement is used for massive industrial placements like big dams, where the heat buildup in a large volume of concrete could create problems.
But Type III cement, the “high early” variety, is common. Residential contractors often call for it when rapid strength gain is important – either because the schedule is tight or because there’s concern about freezing. You can also achieve high early strength by just adding more cement to the mix – say, an extra 100-pound bag per yard – or by using an accelerator like calcium chloride. (In my part of Minnesota, where summers are short and winters are cold, we’ll often do all three: use Type III cement, add an extra bag of cement per yard, and also add some accelerator.)
Air-Entrained Concrete
For most residential work, some amount of air entrainment is essential for long-term freeze/thaw resistance. Air-entrained concrete has billions of microscopic air bubbles mixed into it. If you order air-entrained concrete, your concrete supplier will either use air-entraining cement or add an air-entraining admixture at the batching plant. The mixing action of the truck blends air into the concrete, while the admixture helps the tiny bubbles hold their shape.
When the concrete sets up and hardens, the little air voids give excess water in the concrete space to expand when it freezes. Air-entrained concrete can hold up over many more freeze-thaw cycles than non-air-entrained concrete. By the time fresh concrete reaches a strength of 500 psi (usually within a day or two), the air entrainment is well enough developed that the concrete can withstand freezing.
Entrained air bubbles are very small – mostly between a tenth and a hundredth of a millimeter. Air bubbles larger than a millimeter are not “entrained” air, but “entrapped” air. Entrapped air is not very helpful, and you can mostly get rid of it by rodding, spading, or vibrating the wet concrete as you pour. That consolidation process still leaves the little entrained air bubbles intact.
In Minnesota, we commonly use 6% or even 8% air in a slab designed for outdoor use, or when a freeze might hit before a basement is closed in. A 2% or 3% entrained-air mix is a good precaution, even in moderate climates.
If you think your slab won’t ever see freezing, you can do without air entrainment altogether. But the lubricating effect of the little bubbles in the wet mix, which acts to make the concrete more workable with a lower water content, is a good enough reason by itself to use air-entrained concrete most of the time.
Air entrainment affects the art of finishing slabs. The tiny air bubbles increase the buoyancy of the paste, and slow the upward movement of bleed water. This can influence your finish timing: Where you’d ordinarily wait for bleed water to evaporate before troweling the surface, you may not even see bleed water on an air-entrained slab. Don’t be too quick to jump on the slab, though: That water’s still there under the surface, and some of it needs to evaporate out. Otherwise, you can trap the water under a hard-troweled skin, where it may build up and cause scaling later. If you’re just broom-finishing, no problem, of course.
The Importance of Water
All of concrete’s ingredients are important to its quality. Your supplier, not you, has to worry about providing good quality cement, sand, and gravel. But one big factor is in your hands – the amount of water in the mix. When you holler out “Add water!” during a pour, you really change the concrete. Restrain yourself: Limiting the water content is crucial for strong, hard, durable concrete, and to reduce shrinkage and cracking.
Water/cement ratio. As engineers will tell you, the water/cement ratio is the weight of the water per yard in the mix divided by the weight of cement per yard. Concrete’s water/cement ratio is usually somewhere between .4 and .6.
As an example, let’s take an average yard of concrete, a typical five-bag residential mix. If we start with 30 gallons of water per yard, which weigh 8.33 pounds per gallon, the water weighs about 250 pounds per yard. Five bags of cement per yard, at 94 pounds to the bag, works out to about 470 pounds of cement per yard. Divide 250 by 470, and we find that this typical residential concrete mix has a water/cement ratio of .53 – that is, before the contractor yells out, “Add 20 gallons!”
If you add 20 gallons to a 10-yard load, that’s 2 gallons per yard, or 16.66 pounds. Your water/cement ratio will go up to .56 or .57 – and your concrete will be weaker.
Strength losses. As a rule of thumb, for every gallon per yard of water added on the job, you lose at least 200 psi in strength. If you add 20 gallons to a 10-yard load – 2 gallons per yard – you are probably losing 400 to 500 psi.
Shrinkage. Besides reducing the structure’s ultimate strength, adding water on site will also increase the total amount of shrinkage.
Of the water in a concrete mix, just 25% to 30% is necessary for the hydration reaction. The rest of the water is only there to make the concrete workable. Any water that isn’t involved in the hydration reaction either evaporates (25% to 30%), or remains indefinitely within the pore spaces in the concrete (40% to 50%). Most of the extra water you may add on site will quickly bleed and evaporate out. As the water leaves, the concrete shrinks; as the concrete shrinks, it cracks.
Some cracking of concrete is unavoidable, as we all know. But cracking is directly related to the water content of the mix. More added water means more and bigger cracks. That’s another reason to add as little water as you can.
Reduced density, increased porosity. Not all the water that evaporates out of concrete results in shrinkage. If the concrete sets up and hardens before the water comes out, it won’t typically shrink and crack as much; instead, it will be less dense and more porous. That’s because when you add a lot of water to concrete, you increase the volume; but when the water dries out, all it leaves behind is air.
After oven drying, all of these samples weigh the same amount; but as you can see, the ones with more water are larger. The difference is air – and air doesn’t have a lot of strength. The sample on the left is probably 8,000-psi concrete, but the low-density sample at the right is probably 1,800 psi. The center samples are where most residential concrete is, probably somewhere between 3,000 and 4,000 psi.
Moisture Problems
Besides being weaker, porous concrete made with a high water/cement ratio will end up being more permeable. As concrete is hardening, the cement and the aggregate sink as the bleed water rises. Water flows around the particles and through the sand and cement paste, leaving behind a lot of little voids and capillaries in the hardened concrete (). After the concrete cures, those capillary voids let water and vapor move through finished slabs and walls, which can add to moisture problems.
Most basements experience a certain amount of dampness: at first from water evaporating out of the concrete itself, and later from moisture in the ground moving through the concrete via capillary action. Both moisture loads are directly related to the proportion of water in the mix.
Evaporation. If an average yard of concrete contains 32 gallons of water, and 28% of that evaporates, 9 gallons of water per yard is going to come out of a typical foundation. From the footings, walls, and slab in a big basement, something like 400 to 700 gallons of water vapor will enter the house. That’s a lot of moisture – and the wetter the concrete you pour, the more moisture there will be.
It takes about three years for all of that vapor to come out of the concrete, but the bulk of the evaporation is a first-year concern. About a third comes out in the first 30 days – probably before the house is even finished. The next third comes out in the first year after the pour, so by the end of the first year two-thirds of the water is out. Right when a house is built, if you stud out the basement walls and slap up insulation and poly, you’ll get moisture within the wall. You’d be wiser to let the basement dry out for a while first.
Ground moisture. Unfortunately, water in the poured concrete isn’t the only source of moisture in basements and slabs. All over the country, ground moisture moving through foundation slabs is a common problem.
As the water moves through the slab, it brings up various chemical salts from
the unhydrated cement. The water evaporates and leaves the salt behind as a white fuzzy dust. This material can build up behind a membrane and debond many types of coatings. If rising moisture exceeds about 3 pounds of water per 1,000 square feet over a 24-hour period, it exceeds the breathing ability of many floor coverings: Such moisture can build up and ruin the adhesion of vinyl flooring and tile.
If you pour a stiff mix, the concrete should be watertight. But vapor permeability, though reduced, will still exist. So good concrete alone isn’t the total solution to ground moisture problems: Proper grading, surface drainage, waterproofing, and perimeter drainage are also important (see the related article, ).
How To Do a Slump Test
On site, the accepted way to gauge and control water content is the slump test. To do the test properly, use a standard cone 12 inches high, 4 inches wide at the small end, and 8 inches wide at the big end. You fill the cone up about 1/3 full, rod it 25 times, fill it up to 2/3 full, rod it 25 more times, and then finally fill it to the top and rod it again. Then you remove the cone and measure the slump from the top down.
Although the slump test is basic concrete practice, not everyone understands it. I’ve even seen guys measure from the bottom of the pile of concrete up instead of from the top of the cone down. Then they’ll say, “I’m doing a 2-inch slump concrete.” If they understood the test they’d know they have a 10-inch slump, not a 2-inch slump. That’s a pretty wet mix.
On site you have to make a practical compromise between workability and maximum strength. In the American Concrete Institute (ACI) Committee 332, which I belong to, we’re creating updated standards for residential concrete, and have spent a fair amount of time discussing slumps. Some of the ready-mix guys have argued for the 4-inch slump, but 4-inch-slump concrete is pretty tough material to pour, unless you are going to pump it. All the poured-wall guys wanted to go to a 7-inch slump. In the end, we worked out a compromise: a 4-inch to 6-inch range.
Concrete work is heavy labor, and there’s an understandable desire to pour a higher slump, just to make the concrete more flowable. Suppose you go ahead and increase the slump by adding water? As a rule, it takes a gallon or more of water per yard to add about an inch to your slump. So to increase the slump from 5 inches to 9 inches, you’d need to add about 4 or 5 gallons. That could reduce the strength of your finished concrete by as much as 1,000 psi.
If you need such flowable concrete, you should get your supplier to teach you about superplasticizers. If you start with a 5-inch slump and then superplasticize it, you can get an 8-inch or 9-inch slump that is relatively easy to work with. And because you don’t have to add water, you’ve maintained good strength.
Now, you can do a good job with very flowable concrete if you move the chute and puddle the concrete where it is intended to be. But if you dump a whole lot in one corner and then pull it along the form with hoes, the aggregate starts coming out of the flow and separating from the cement. Where there’s a lot of gravel in one place and not enough cement, the result is honeycombing. A result like this usually means that someone tried to make the concrete flow too far. You can also see the results here as visible lines in the wall.
You’ll also get lines like that if there’s a delay between one lift of concrete and the next, especially if you pour a wall in hot weather. Once you start a wall pour, it’s best to keep the concrete coming at a steady rate, and not allow part of the wall to set up before the next lift is placed on top of it.
You should also consolidate each lift in a wall as you place it. Very flowable concrete can be consolidated by rodding it with a steel rod; stiff concrete is better consolidated with a vibrator. But if you have a high-slump mix, over-vibrating can create problems: It causes the gravel and sand to segregate out from the cement, and increases the pressure on the forms, which may cause you to pop a pin on a snap-tie, causing the wall to “pillow” out. One popped pin is more of an aesthetic problem than a structural problem, but too much pressure on the forms could cause a blow-out.
Vibrating is appropriate when you use wood forms or steel forms. Contractors with aluminum forms have to be very careful vibrating, because the aluminum forms are somewhat deflectable and may pillow from the pressure.
Use the vibrator to consolidate the concrete in place and get the big air bubbles out of it. Don’t use the vibrator to move the concrete from one spot to the next – that typically creates segregation. And while it’s not a big deal if the vibrator bumps up briefly against the steel (in fact, this probably improves the steel-to-concrete bond), you don’t want to deliberately vibrate the steel by holding the vibrator to the rebar. That could actually weaken the bond between steel and concrete.
There’s a lot more to learn about building with concrete – things like how to use admixtures, how to control cracking, how to deal with cold or hot weather, how to cure concrete, and when, how, and where to place reinforcing steel. But if you start with the basics – choosing the right cement, using large, well-graded aggregate, keeping the water content down, and placing and consolidating carefully – you’re off to a good start.